This is a re-post of an article published May 19, 2019 by Vitaly Alekseevich Kirkinsky at REGNUM in honor of the 30th anniversary of the announcement of cold fusion.
Details: https://regnum.ru/news/innovatio/2631134.html
Any use of materials is allowed only if there is a hyperlink to REGNUM news agency.
A remote report by the leading technologist of the Institute of Geology and Mineralogy of the Siberian Branch of the Russian Academy of Sciences, named after Academician V. S. Sobolev, Doctor of Geological and Mineralogical Sciences, Corresponding Member of the Russian Academy of Natural Sciences, Vitaly Alekseevich Kirkinsky presented “Cold nuclear fusion and transmutation of elements: experiments, theory, patents, natural manifestations” at the conference “Cold fusion – 30 years: results and prospects”, held in Moscow on March 23, 2019.
* * *
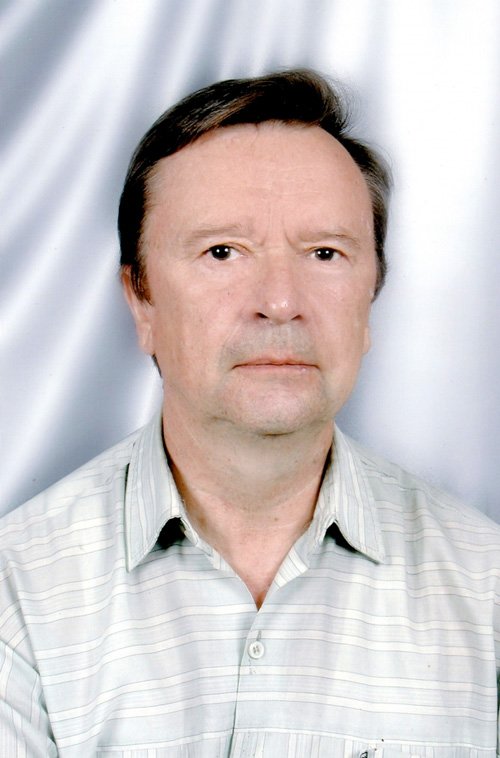
I became interested in cold fusion right after 30 years ago when the radio news of electrochemists Martin Fleishman and Stanley Pons at the University of Utah, USA, was announced on the radio. They argued that during electrolysis of lithium salt solutions in heavy water, a yield of neutrons and excess energy of about 1 watt was observed at the palladium electrode, as well as an increase
in tritium concentration in the solution, which, in their opinion, was caused by nuclear fusion of helium from deuterium. This did not fit into the existing ideas of physicists at all, since such reactions could only be carried out at enormous energies. The opinion was that this data was the result of an error or a fraud. There were very serious arguments in favor of this: no products of nuclear reactions were detected, an increase in the tritium content could be caused by its accumulation upon evaporation of heavy water, and the energy release should have been accompanied by a huge neutron flux.
According to the accepted theory, the implementation of thermonuclear fusion requires temperatures of more than 100 million degrees. The fundamental idea of plasma heating and confinement in toroidal chambers placed in a magnetic field – TOKAMAKs was proposed by academicians A. D. Sakharov and I. E. Tamm 70 years ago. The practical implementation of this idea ran into extreme technical difficulties. According to Academician E.P. Velikhov, more than $ 40 billion has already been spent on these works in our country. Russia is participating in the ITER international fusion reactor development program, $20 billion is planned to be spent on the first stage only. By 2027, it is planned to build an experimental reactor and begin experiments with plasma, which can give the answer – whether it will be possible to create the necessary conditions for thermonuclear combustion. If successful, the test results will be the basis for the project even larger – a demonstration thermonuclear reactor DEMO. The DEMO experience in turn will serve as the basis for the design of the first experimental industrial station. However, even if all the scientific and technical problems in half a century can be solved, there are big doubts about the economic feasibility and safety of obtaining energy in fusion reactors.
Given the enormous cost of the project, the life of the reactors due to the strong neutron flux, judging by the experience of operating less powerful tokamaks, will be only a few months. Neutron-free reactions require even higher plasma temperatures and much more expensive reactors.
According to the technical conditions, the thermonuclear reaction can be maintained only in large-volume reactors. A single filling of the working chamber of the reactor with a volume of 830 cubic meters. meters with a mixture of deuterium and tritium will cost more than a billion dollars. Only due to the decay of radioactive tritium monthly losses amount to more than $ 160 thousand. Tritium requires atomic reactors. Diffusion of deuterium and tritium through the walls of the reactor or microcracks can lead to the formation of an explosive mixture with atmospheric oxygen and the explosion of a reactor with serious consequences.
The possibility of implementing nuclear fusion at low temperatures could open up tremendous prospects for energy.
About a hundred groups around the world tried to reproduce the experiments of Fleischmann and Pons [30]. The most convincing results were obtained in Japan [31–33]. Yoshiaki Arata and Yui-Chang Zhang found an excess heat yield of 200–500 MJ / cm3 and the formation of a significant amount of helium in a deuterated palladium black placed in a closed palladium ampoule, which served as a cathode for 5,000 hours of electrochemical experiments. It should be specially noted that the Helium-3 / Helium-4 ratio in the experimental products was 4–5 orders of magnitude higher than atmospheric. Similar experiments were replicated in the laboratory of the Electric Power Research Institute in the USA [34]. The release of excess heat and its correlation with the release of tritium and helium was confirmed. The ratio of Helium-3 / Helium-4 in the products of the experiments was 44,000 times higher than atmospheric.
These and many other results were not published in peer-reviewed journals, but mainly in the materials of international and national conferences. Official science considered them unreliable. Even 23 years after the first report of a new phenomenon in the obituary about the death of Martin Fleischman in the authoritative journal Nature, it was written:
“… cold fusion is now regarded as one of the most famous cases of what the chemist Irwin Langmuir called pathological science: science of things that aren`t so.”
The main reason for the persistence in ignoring the new scientific direction was the impossibility of a theoretical explanation of the experimental data. As the whole history of the development of science shows, new phenomena are recognized only after the conditions for their reliable reproduction are found and a theoretical explanation is given on the basis of the fundamental laws of nature. Building a theory of the phenomenon is an essential stage of a major discovery. For this reason, the development of the theoretical foundations of the mechanism and kinetics of nuclear reactions in condensed matter at low energies is no less important than the detection and confirmation of anomalous phenomena. For practical use in the energy sector, it is necessary to increase the intensity of nuclear reactions by a factor of millions in comparison with the first experiments, which is extremely difficult to implement without a theoretical understanding of the phenomenon.
Since 1989, more than a hundred works have been published in which the most diverse hypotheses have been expressed about the causes of the “Fleischmann and Pons effect.” Links and their classification is given by us in [2, 5]. Most authors were limited to assumptions made in qualitative form. In a survey [35], the theorists of the United States and Russia concluded:
“Despite considerable efforts, it was not possible to create a theory of cold nuclear fusion that quantitatively or even qualitatively describes experimental results. Models in which it is stated that they have solved this task are far from achieving the goal. ”
At many subsequent international conferences, it was noted that the creation of the theory of nuclear reactions in condensed matter is a task of paramount importance.
Experimenters carried out and still conduct experiments mostly by the inefficient trial and error method. At the 9th Beijing Cold Synthesis Conference in 2003, I asked Martin Fleishman a question; what, in his opinion, is more important for the development of this direction: experiments or theory? He answered briefly: “Both” .
* * *
From the very beginning of our research, we set as the main task the development of the theory of nuclear reactions at low energies, combining this with experiments.
The problem of overcoming the Coulomb barrier is covered in articles published in Europhysics Letters [2, 3], a monograph [5] and a number of articles in International Conference Materials [6, 7, 10–12].
Our model of the mechanism of nuclear reactions is based on taking into account the dynamic screening of proton (deuteron) charges by external electronic orbitals of metal atoms. Both semiclassical and quantum mechanical models were used. Several hundred thousand numerical experiments were carried out using molecular dynamics methods at random initial positions of deuterons during their diffusion in the crystal structures of a number of metals, which showed how close they are to each other. It turned out that, although the average distance between them is approximately the same as in the D2 molecule – 0.74 Ǻ, several percent of the pairs come closer to a distance of less than 0.1 Ǻ, up to 0.01 Ǻ. At such distances, nuclear fusion occurs due to the tunnel effect, which is calculated according to the formulas generally accepted in quantum mechanics. Calculations using these models for the first time allowed us to obtain quantitative data on the probability and rate of nuclear reactions of hydrogen isotopes in a number of metals: palladium, titanium, lanthanum, alpha- and gamma-iron [5–8, 11, 12, 14].
Together with the theoretical physicist of Altai State University, candidate of physical and mathematical sciences A. I. Goncharov, we performed a computer simulation of the behavior of hydrogen atoms in a medium of free electrons in metals [13]. A previously unknown phenomenon has been discovered: the formation of unsteady complexes of protons or deuterons with orbits of electrons rotating around them in varying size and shape. In size, they are 3–4 orders of magnitude smaller than a hydrogen atom and only one order larger than a neutron. We called them miniatoms or quasineutrons. Due to their electrically neutrality, in a short time of their existence, they can freely move in the crystalline structures of metals and approach the nuclei of hydrogen or metal isotopes at distances at which nuclear interaction occurs due to the tunnel effect. This solves the key problem of overcoming the Coulomb barrier. The calculated reaction rate between deuterons in palladium deuteride taking into account the formation of miniatoms is 6 orders of magnitude higher than previously obtained on the basis of the model of dynamic deformation of electronic orbitals.
Our calculations allowed us to find ways to intensify nuclear reactions of deuterium in the crystal structure of metal hydrides. It was possible to find a nontrivial and effective way to intensify nuclear interaction due to isostructural phase transitions, the probability of overcoming the barrier at which increases significantly, which increases the rate of nuclear fusion by several orders of magnitude.
The reasons for the extremely strong (tens of orders of magnitude) attenuation of neutron and hard gamma radiation during nuclear reactions in metal hydrides and deuterides at low temperatures are justified in comparison with thermonuclear processes in plasma. This is due to the mechanism of nuclear reactions occurring through the intermediate stage of the formation of miniatoms. The characteristic features of such reactions in metal hydrides (deuterides) and their effect on radioactive radiation are considered in [21]. It has been shown that nuclear fusion energy is released mainly in the form of softer – X-ray radiation, which, when absorbed in metals, fuel, reactor and cooling system leads to their heating. This is a very practical feature of nuclear reactions in condensed matter, since protection against x-ray radiation with the help of screens is not difficult and is well developed in scientific and medical devices.
The theoretically calculated emission of excess energy in the process of the α-β transition in palladium deuteride was verified by us together with the thermochemical measurement expert V. A. Drebushchak in experiments on the SETARAM DSK-III scanning calorimeter using a specially developed technique. The results of eight series of experiments showed that during the sorption-desorption of deuterium in a fine-crystalline palladium powder, an excess energy of more than 1 W per gram of palladium deuteride is released, while in similar experiments with a light isotope of hydrogen, no anomalous effects were observed. These results were published by us in the Europhysics letters [4] and in the materials of the international conference [9].
Based on the theoretical and experimental studies, a method and device for energy production were developed, for which two Russian patents [26, 27], Eurasian and European patents [28, 29], each of which includes more than 20 private inventions, were obtained.
Their main features are the use of nanopowders of specially selected metals and intermetallic compounds, which, when saturated with deuterium or ordinary hydrogen, undergo isostructural transformations with a change in composition with a change in temperature or pressure.
In Fig. 1 shows a diagram of the device according to patent [26] with a priority date of August 3, 1992.
The installation includes two interconnected steel vessels 1 and 2 with valves 3 and 4 and pockets in which electric heaters 5, 6 and thermocouples 9 and 10 are placed. Outside the vessels there are copper tubes 7 and 8 with cooling fluid. A fine-crystalline metal (Me) is placed inside the vessels, whose hydrides or deuterides undergo an isostructural transition with temperature. Compressed hydrogen, deuterium or their mixture is fed from the connected cylinder 16 to one of the vessels until complete saturation, then the heater is turned on and the valve opens to connect to the second vessel, outside of which cooling water is passed. After a while, the heater of the second vessel turns on, and the process goes in the opposite direction. The cycles of sorption-desorption are repeated many times.
In Fig. 2 shows a diagram of a deuterium heat generator according to patents [27, 28] together with a system for measuring energy balance.
Designations in Fig. 2: 1 – the inner cylinder of the reactor, 2 – the outer cylinder of the reactor, 3 – the cooling casing, 4 – the working volume with the working substance, 5 – shutter, 6 – pressure nut, 7 – dust filters, 8 – locking seal block, 9 – flange joints with a vacuum system and a shut-off valve, 10 – thermal insulation, 11 – heating elements, 12 – coolant, 13 – seals, 14 – pressure nut of the cooling sleeve, 15 – supply and control system for the flow of coolant, 16 – thermocouple measuring unit, 17 – thermostat combined thermocouples s, 18 – power supply, 19 – transformer, 20 – thermocouples, 21 – thermocouple temperature sensor of the liquid entering the heat exchanger, 22 – thermocouple temperature sensor of the liquid leaving the heat exchanger, 23 – Watt-hour electric meter of active energy.
A general view of the manufactured installation is shown in Fig. 3
32.7 g of specially prepared fine crystalline palladium with a particle size of 20 to 100 nm were placed in a 308 cm3 volume heat generator reactor. After evacuation to ~ 1 Pa, from 700 to 2600 ml of gaseous deuterium obtained from heavy water were introduced into the reactor. Measurements were carried out both at constant temperature and pressure, and with cyclic temperature changes from 50º to 600ºC. The energy consumed was measured by the voltage and current strength in the heater, and the released energy was calculated by the heat capacity and the mass of water heated in the heat exchanger. The results of experiments on the dependence of excess energy on temperature are presented in the graph (Fig. 4) [18].
The relative excess energy averaged ~ 23% with maximum values up to 35% of the expended energy, which corresponds to the emitted power of ~ 20 Watts per gram of palladium or 1 kW per gram of deuterium. The maximum excess power was ~ 600 watts. The total amount of excess energy released is ~ 100 MJ, which is 2500 times higher than the energy of possible chemical reactions in the reactor. This proves that excess energy is due not to chemical, but to nuclear processes. The energy release, which is 25–35% higher than that consumed, was confirmed in a series of experiments with cycles of heating and cooling the reactor.
Evidence of nuclear reactions in the reactor is an increase in neutron and gamma radiation fluxes when the temperature rises to 400ºC and decreases to the background level during cooling (Fig. 5 and 6) [21].
The measured increase in radioactive radiation does not exceed variations in the natural cosmic background, but the possibility of reproducibly changing their level depending on temperature proves that nuclear reactions occur in the reactor.
The observed intensity of radioactive radiation is many orders of magnitude lower than in thermonuclear reactions in plasma for the equivalent release of total energy, which has been repeatedly noted in all studies of cold nuclear fusion. Nevertheless, it should be said that safety issues, especially when working with plasma plants for cold nuclear fusion, require further serious study.
Even more convincing evidence of nuclear reactions was obtained by examining the contents of the reactor after a series of 65 experiments.
The analysis of the initial palladium and products obtained after the whole series of experiments was carried out by two methods of atomic emission spectral analysis at the Institute of Geology and Mineralogy of the SB RAS. In the first of them, developed by VMK-Optoelectronics, the “wake-up-blowing” method at the Potok installation with electric arc excitation, the samples were mixed with especially pure graphite at a ratio of 1:50 and after grinding in a mortar they were fed into an electric arc. Five parallel samples were measured by comparing the intensities of 2–3 spectral lines with standards of known composition.
In another method, atomic emission spectral analysis with inductively coupled ISP-AES plasma, IRIS used solutions previously prepared by dissolving the test substances. We also used laser mass spectral analysis of MS-AES at the IONH RAS using an EMAL-2 instrument. The isotopic composition of palladium was also determined at the IGM SB RAS by the mass spectral method with inductively coupled ICP-MS plasma.
A comparison of the results of analyzes performed by the methods used allows us to come to the following conclusions [19].
1. During the interaction of gaseous deuterium with a number of elements – impurities in the initial palladium: Li, Be, B, C, F, Mg, Si, S, K, Ca, Ti, V, Fe, Co, Ni, Zn – their transmutations were observed that are described by generalized nuclear reactions:
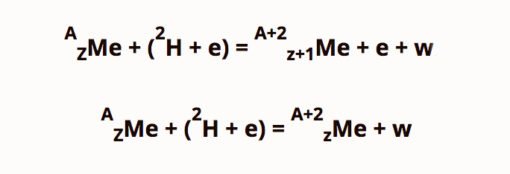
with the release of significant energy w, calculated from the increase in mass defect (Table 1).
2. For 15 elements in which a similar reaction would lead to a decrease in mass defect: Ge, As, Y, Cd, Sn, Sb, La, Nd, Sm, Eu, Gd, Tb, Dy, Ho, Er, Tm, Yb , Lu, Pt, Hg, Pb, Bi, Hf, Ta, a change in the content of elements within the error of spectral analysis methods does not occur.
3. A significant (by two orders of magnitude) increase in the silver content in the product of experiments is most likely due to the reaction of palladium isotopes with high-energy protons — products of a nuclear fusion reaction from deuterons.
4. The isotopic composition of the palladium product of the experiments within the accuracy of the analysis of ICP-MS (± 1%) is identical to the original.
5. The estimate of the energy released during nuclear reactions of the synthesis of helium isotopes from deuterium and due to the transmutation of impurity elements approximately corresponds to the total energy released in the entire cycle of experiments.
The geological evidence of nuclear reactions of hydrogen in the core of the Earth are: high heat flux from a nucleus of 13 ± 3 TW recorded by geophysicists, unexplained by known causes; abnormal ratios of isotopes of He, S, Fe and others in rocks of deep origin and associated hydrothermals; high contents of heavy Fe isotopes in iron meteorites – the remnants of metal nuclei of asteroids (analogues of planetary nuclei) The energy release in nuclear reactions of hydrogen, observed in experiments, in terms of the mass of the nucleus, is much higher than the heat flux from it, and the current energy estimates of the hydrogen content in the core are sufficient to ensure the total heat flux of the Earth over many billions of years. The melting of silicate rocks caused by the heating and formation of water when hydrogen enters the mantle leads to the formation and rise of giant magmatic masses — plumes, an increase in the Earth’s radius and the breaking of its upper hard shell — the lithosphere into large plates. The arrival of hot magmas to split cracks leads to the formation of areas of elevation of the level of the asthenosphere (partially molten layer) and the sliding of plates from them under the action of gravitational forces. Chips of compression occur in the areas of plate collision and subduction zones are formed — plate immersions or mountain systems are formed during the thickening and deformation of the lithosphere. The mechanism that drives lithospheric plates is discussed in detail in my previously published article and monograph [23, 24]. At that time, the reason for the warming up and expansion of the Earth was unclear. Our subsequent work found that the reason for this is the energy released during nuclear reactions of hydrogen in the Earth’s core. The rise of large plumes in the continental regions, which originated on the border with the core, causes outpouring of basaltic magma, an example of which are gigantic Siberian traps in thickness. The processes occurring under the influence of nuclear reactions in the Earth’s core are ultimately the root cause of the origin of many magmatic and hydrothermal ore deposits, in particular nickel, platinum, palladium, gold and others. The reactions of cold nuclear fusion and transmutation of elements are the main energy source of global geological processes.
Theoretically and experimentally established, as well as confirmed by natural facts, the possibility of synthesis and transmutation of elements not only in stars, but also in terrestrial conditions, is of fundamental importance for geochemistry and cosmochemistry.
Currently, studies of nuclear reactions at low energies are intensively conducted in many countries of the world, hundreds of articles and dozens of patents have been published, and international and national scientific conferences are held annually. Unfortunately, this branch of science in our country has not yet received government support. Work on this topic is associated with risk, so it was not included in the research plans and was not funded. The publication of works that run counter to traditional ideas is extremely difficult, and in Russian magazines – until recently, it was actually banned. The lack of articles in leading journals was the reason for rejecting applications at the RFBR – an alternative source of funds for basic research. For 30 years, not a single cold fusion project has been supported.
It is also worth noting that the formation of this direction coincided with two decades of perestroika, which very seriously affected the financing of science. Private investors are not interested in investing in projects that do not guarantee quick returns. For these reasons, we conducted expensive studies at our own expense. Almost all groups working on this subject were in the same position. Many of them disbanded, and some researchers went abroad. The continuation of such a scientific and technical policy will lead to a technological lag in our country. The success of the Russian enthusiasts will not be enough for development. Russia will have to pay to foreign patent holders for each kilowatt-hour of energy produced by the new technology.
IA REGNUM, providing authors with the opportunity to popularize their developments, makes an important contribution to the development of this breakthrough direction.
Details:
1. Kirkinsky V. A., Novikov Yu. A. 1997. The problem of nucleosynthesis in geological processes. In the book. “Earth sciences on the threshold of the 21st century: new ideas, approaches, solutions”, Moscow, Scientific World, p. 85.
2. Kirkinsky V. A., Novikov Yu. A. 1998. Theoretical modeling of cold nuclear fusion. Novosibirsk, 48 p.
3. Kirkinskii V. A., Novikov Yu. A. 1999. A new approach to theoretical modeling of nuclear fusion in palladium deuteride. Europhysics Letters, v. 46, No. 4, pp. 448−453.
4. Kirkinskii V. A., Drebushchak V. A., Khmelnikov A.I. 2002. Excess heat release during deuterium sorption-desorption by palladium powder palladium deuteride. Europhysics Letters, v. 58, No. 3, pp. 462−467.
5. Kirkinskii V. A., Novikov Yu. A. Theoretical modeling of cold fusion. Novosibirsk, Novosibirsk State University, 2002, 105 p.
6. Kirkinskii V. A., Novikov Yu. A. 2002, Hydrogen Isotopes of Numerical Computation. Experiment in Geosciences, v. 10, No1, pp. 51−53.
7. Kirkinskii V. A., Novikov Yu. A. 2003. Numerical calculations of cold fusion in metal deuterides. In the book: “Condensed Matter Nuclear Science” (Proceedings of the ICCF-9, ed. By Xing Z. Li), pp. 162−165
8. Kirkinskii V. A., Novikov Yu. A., 2003. Freedom of the Earth’s interior. In the book: “Condensed Matter Nuclear Science” (Proceedings of the ICCF-9, ed. By Xing Z. Li), 166−169, 2003.
9. Kirkinskii V. A., Drebushchak V. A., Khmelnikov A.I. 2003. Experimental evidence of heat output during deuterium sorption-desorption in palladium deuteride. In the book: “Condensed Matter Nuclear Science” (Proc. Of the ICCF-9, ed. By Xing Z. Li), pp. 170−173.
10. Kirkinskii, V. A., Novikov, Yu. A. 2004. Modeling of dynamic screening effects in solid state. Europhysics Letters. Vol. 67, N 3, pp 362−367.
11. Kirkinskii V. A., Novikov Yu. A., 2006. Calculation of nuclear reaction probability in a crystal lattice of lanthanum deuteride. In the book “Progress in condensed matter nuclear science.” Editor A. Takahashi, World Scientific Publ. Co., Proc. of 12th Conference on cold fusion.
12. Kirkinskii V. A., Novikov Yu. A., 2006. Calculation of nuclear reaction probabilities in a crystal lattice of titanium deuteride. In the book “Condensed Matter Nuclear Science”. Editors: P. Hagelstein and S. Chubb. World Scientific, Proc. of the ICCF-10, pp. 681–685.
13. Goncharov A I., Kirkinskii.V. A., 2006. Theoretical modeling of electron flow action on probability of nuclear fusion of deuterons. In the book “Progress in Condensed Matter Nuclear Science”, Editor A. Takahashi. World Scientific Proceedings of 12th conference on cold fusion.
14. Kirkinskii V. A., 2008. Estimation of geofusion probability. In the book: Proceedings of the 13th International Conference on Condensed Matter Nuclear Science (ICCF 13), Moscow, pp. 674−678.
15. Kirkinskii V. A., Khmelnikov A. I., 2008. Setup for measuring of energy balance at interaction of metals and hydrogen isotopes gas at high temperatures and pressures Proc. of the 13th International Conference on Condensed Matter Nuclear Science (ICCF-13), Moscow, p. 43–46.
16. Kirkinsky V. A., 2015. Experimental evidence of nuclear reactions in the Earth’s core. Proceedings of VESEMPG-2015. P.270-275.
17. Kirkinsky V. A., 2015. Nuclear hydrogen reactions as a source of energy for the Earth’s core. Proceedings of VESEMPG-2015. P.276−281.
18. Kirkinsky V. A., Khmelnikov A. I., 2016. Results of measurement of excess energy in a deuterium heat generator. Materials of the 22nd Russian Conference on Cold Transmutation of Cores of Chemical Elements and Ball Lightning, p. 105−115, Moscow.
19. Kirkinsky V. A., Khmelnikov A. I., 2016. Transmutation of elements in a deuterium telogenerator: preliminary results. Materials of the 22nd Russian Conference on Cold Transmutation of Cores of Chemical Elements and Ball Lightning, p. 116−123. Moscow.
20. Kirkinsky, VA, 2016. Nuclear reactions of the synthesis and transmutation of elements in the Earth’s core, Proceedings of the 22nd Russian Conference on Cold Nuclear Transmutation of Chemical Elements and Ball Lightning, p. 125−135, Moscow.
21. Kirkinsky V. A., 2016. Neutron and gamma radiation in a deuterium heat generator in connection with the problem of the mechanism of nuclear reactions at low energies Materials of the 24th Russian Conference on Cold Transmutation of Nuclei of Chemical Elements and Ball Lightning, p. 91−100, Moscow.
22. Kirkinsky V. A., Natural evidence of nuclear reactions of synthesis and transmutation of chemical elements in the Earth’s core. Materials of the 25th Russian Conference on Cold Transmutation of Cores of Chemical Elements and Ball Lightning, 2019 (in press), Moscow.
23. Kirkinsky V. А., On the physicochemical mechanism of global tectonic processes. Geology and Geophysics, 1985, No. 4, p.3−14.
24. Kirkinsky V. A., The Mechanism and Cyclicity of Global Tectogenesis. 1987, Novosibirsk, Science, 71 p.
25. Kirkinskii V. A., 1994. Tritium, helium and free neutrons. (The method of obtaining energy, as well as helium, tritium and free neutrons and devices for its implementation). International application published under the Patent Cooperation Treaty (PCT). PCT / RU93 / 00174 International Application Number. MKI G21 B1 / 00 G21 G4 / 02. International publication number WO 94/03902. 17.02.94, 30 s.
26. Kirkinsky V. A., 1996. Patent of the Russian Federation No. 2 056 656 for the invention “Method for producing free neutrons”. Priority date August 3, 1992 Published in the bulletin “Inventions, Trademarks” March 20, 1996, No. 8, part II, p. 267-268.
27. Kirkinsky V. A., Khmelnikov A. I., 2002, Device for generating energy. The patent of the Russian Federation № 2 195 717. The bulletin “Inventions, trademarks”, № 26.
28. Kirkinsky VA, Khmelnikov AI, 2006. Device for generating energy. Eurasian Patent No. 006525 In 1, Int. Class. G21B / 00, date posted 2006.02.24.
29. Kirkinskii V. A., Khmelnikov A. I., 2009. Energieеrzeugungseinrichtung (Power Producing Deviсe) Europaische Patentschrift 1 426 976 B1, Int. Cl. G21B 1/00 Publikation Date 12/23/2009, Patentblatt 2009/52.
* * *
References
30. Fleishman M., Pons S., J. Electroanal. Chemistry, 1989, vol. 261, p. 301-308.
31. Arata Y. and Zhang Y. Ch., Proc. Japan Academ., 1996, vol. 72, ser. B, p. 179−184.
32. Arata Y. and Zhang Y. Ch., Proc. Japan Academ., 1997, vol. 72, ser B, p. 1-6.
33. Arata Y. and Zhang Y. Ch., Proc. Japan Academ., 1999, vol. 75, ser B, p. 76, p. 281.
34. McCubre M., Crouch-Baker S, Hauser A. K. et al. In Proc. ICFF-8, 2000, Lerichi, Itali, 2001.
35. Chechin V. A., Tsarev V. A., Rabinovitz M., Kim G.E., Int. J. Theor. Phys., 1994, v. 33, p. 617−670.
36. Rossi A., US Patent 2014/0326711 A1.
37. Levi G., Foschi E., Hoistad B., Pettersson R., Tegner L. and Essen H. Observation of abundant heat production from a reactor device and of isotopic changes in the fuel.
38. Parkhomov A. G. The journal of emerging fields of science, 2015, v.3, no. 7, p. 68−72.
This is a re-post of an article published May 19, 2019 by Vitaly Alekseevich Kirkinsky at REGNUM and presented the 30th anniversary of the announcement of cold fusion.
